Clinical record
A 39‐year‐old woman underwent bilateral sequential lung transplantation for fibrotic hypersensitivity pneumonitis in May 2022. Her immunosuppression treatment included prednisolone, tacrolimus and mycophenolate. She received one dose of Comirnaty (Pfizer) pre‐transplant and tixagevimab–cilgavimab in June 2022.
Her first severe acute respiratory syndrome coronavirus 2 (SARS‐CoV‐2) infection was diagnosed on 14 December 2022 and she was treated with molnupiravir for five days. On Day 11 of illness, she presented to hospital in respiratory distress with hypoxia (SpO2; 73% on room air). SARS‐CoV‐2 RNA was detected by polymerase chain reaction (PCR) with a cycle threshold of 21.8 (cobas SARS‐CoV‐2 and Influenza A/B, Roche). A chest computed tomography scan demonstrated extensive bilateral ground‐glass opacification (Box 1). She required intensive care unit admission for high‐flow nasal oxygen (FiO2 60%, flow rate 50 litres) and received tocilizumab, ten days of remdesivir, increased prednisolone, and piperacillin–tazobactam. Three days of pulsed methylprednisolone was prescribed for possible transplant rejection. Despite clinical improvement, SARS‐CoV‐2 RNA remained detectable with a cycle threshold of 16.2 (VIASURE SARS‐CoV‐2, flu and RSV, Certest Biotec) and SARS‐CoV‐2 was isolated from a cell culture.1 Whole genome sequencing identified Omicron BR.2 (variant of concern B.1.1.529) lineage, with molnupiravir‐associated mutational signatures,2 (sequence available on GISAID [Global Initiative of Sharing All Influenza Data]; 26/12/22: hCoV‐19/Australia/NSW_ICPMR_40135/2022 and 03/03/23: hCoV‐19/Australia/NSW_ICPMR_43136/2023).
The patient was transferred to a high acuity respiratory ward after three weeks, but over the ensuing six weeks, became severely deconditioned and continued to require high‐flow nasal oxygen (FiO2 30–35%, 35 litres). Chest imaging was stable, demonstrating fibrosis but minimal progressive inflammation. The persistent detection of SARS‐CoV‐2 RNA and isolation of SARS‐CoV‐2 by culture (Box 2) from upper respiratory tract samples prevented participation in enhanced inpatient pulmonary rehabilitation beyond her single room, as per local infection prevention guidelines and hospital policy.3 The policy, based on national guidelines,3 dictated that for coronavirus disease 2019 (COVID‐19) de‐isolation, immunocompromised hosts need to be 21 days post‐infection, asymptomatic, and without detectable SARS‐CoV‐2 RNA. In individuals with persistent RNA detection, a cycle threshold greater than 30 with either positive spike antibody, negative rapid antigen test (RAT) or culture is sufficient for de‐isolation.
The patient received a further dose of tixagevimab–cilgavimab, regular intravenous immunoglobulin and ten further days of remdesivir. Repeat whole genome sequencing did not identify infection with another SARS‐CoV‐2 lineage nor genomic markers of antiviral resistance.4 Convalescent plasma was not available and administration of virus‐specific T‐cells was deemed too high risk due to the patient's compromised immune system and fragile respiratory status. The patient received further remdesivir and molnupiravir and was weaned to 1–2 litres of oxygen (FiO2 24–28%). At this point, the patient had been in isolation for 16 weeks as per the de‐isolation protocol, requiring dedicated psychosocial input. A novel clearance strategy was subsequently implemented with twice weekly PCR and extended viral culture tests. De‐isolation criteria were modified to require two negative cultures, irrespective of cycle threshold value. A decision was made to de‐isolate at 25 weeks when no viable virus was isolated after 98 days of diagnosis, and imaging was stable. Coincidentally, PCR cycle threshold was persistently greater than 30. Following rehabilitation, she was discharged after seven months of illness.
Discussion
Persistent viral shedding is increasingly recognised in COVID‐19, with recent data suggesting the median duration of viable SARS‐CoV‐2 in immunocompromised hosts is four weeks5 in contrast to immunocompetent hosts where SARS‐CoV‐2 is rarely isolated after ten days.6 Up to 7% of solid organ transplant (SOT) recipients shed virus for more than 30 days.7 Although relatively common, there is limited data to guide de‐isolation of immunocompromised hosts, impacting infection control, allied health, and resource use.6 Treatment options are also limited, with molnupiravir no longer recommended for treatment in SOT recipients, due to concerns regarding the promotion of viral escape and evolution.2 Further, logistical difficulties in administering outpatient remdesivir, and nirmatrelvir–ritonavir in the context of drug–drug interactions have limited their use. Additionally, this and other novel Omicron strains have been increasingly resistant to sotrovimab and although tixagevimab–cilgavimab retains some in vitro activity, neutralisation is reduced.8
Existing COVID‐19 diagnostics, including PCR cycle threshold values and culturable virus, are imperfect surrogates for infectivity.9 Although there is decreased likelihood of isolating replication‐competent SARS‐CoV‐2 from samples with cycle threshold values greater than 30, correlation in immunocompromised hosts with protracted shedding is less established. Moreover, there is variability among immunocompromised hosts, with SOT recipients less likely to shed viable virus than haematological transplant recipients.10 Heterogeneity in this patient population might be further influenced by differences in immunity following infection or vaccination.11 In immunocompromised hosts with persistent shedding, RATs may be a useful clearance test as the negative predictive value increases from 48% to 92% after 20 days,5 and as a collective approach, PCR, viral culture and RATs can be useful in complex cases. De‐isolation strategies vary across Australia, with national guidelines potentially leading to long periods of isolation.3,12 The exclusion of immunocompromised hosts from many studies on which these recommendations are based means it is difficult to extrapolate data to this cohort.11 To mitigate this, individual patient assessment by multidisciplinary teams to determine timing for de‐isolating immunocompromised hosts with persistent SARS‐CoV‐2 detection is practised at some transplant centres. Contemporaneous resources on vaccination regimens, immunosuppressant modulation and updated de‐isolation guidelines have since been released, reflecting the changing requirements for immunocompromised hosts with COVID‐19 requiring hospitalisation.12
Ultimately, this case highlights the difficulty in managing protracted SARS‐CoV‐2 infection in lung transplant recipients, emphasising the need for patient‐specific therapeutic management and adaptable approaches to infection control in complex immunocompromised hosts.
Lessons from practice
- Protracted viral shedding is relatively common in immunocompromised hosts and existing de‐isolation guidelines may result in impractical long periods of isolation.
- Existing coronavirus disease 2019 (COVID‐19) diagnostics, including rapid antigen tests, polymerase chain reaction cycle threshold value and culturable virus, are imperfect surrogates for infectivity; however, collectively can play a role in assessing prolonged viral shedding.
- Pragmatic de‐isolation strategies are required for patients with prolonged SARS‐CoV‐2 infections.
Patient consent
The patient provided written consent for publication.
Box 1 – Chest computed tomography scan on admission (left) demonstrates extensive bilateral ground glass changes, particularly in mid‐zones and perihilar regions (blue arrows), concerning for infection. Scan pre‐de‐isolation (right), with extensive interstitial and traction bronchiectatic changes (red arrows) indicative of fibrosis as opposed to ongoing infection
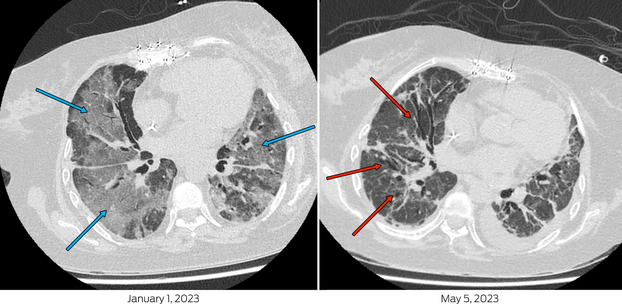
Provenance: Not commissioned; externally peer reviewed.
- 1. Basile K, McPhie K, Carter I, et al. Cell‐based culture informs infectivity and safe de‐isolation assessments in patients with coronavirus disease 2019. Clin Infect Dis 2020; 73: e2952‐e2959.
- 2. Sanderson T, Hisner R, Donovan‐Banfield I, et al. A molnupiravir‐associated mutational signature in global SARS‐CoV‐2 genomes. Nature 2023; 623: 594‐600.
- 3. Australian Government Department of Health and Aged Care, Australian Centre for Disease Control. Coronavirus Disease 2019 (COVID‐19) CDNA National Guidelines for Public Health Units. Version 8.0. Australian Centre for Disease Control, 2024.
- 4. Fong W, Rockett RJ, Agius JE, et al. SABRes: in silico detection of drug resistance conferring mutations in subpopulations of SARS‐CoV‐2 genomes. BMC Infect Dis 2023; 23: 303.
- 5. Kang SW, Kim J, Kim JY, et al. Virological characteristics and the rapid antigen test as deisolation criteria in immunocompromised patients with COVID‐19: a prospective cohort study. J Med Virol 2023; 95: e29228.
- 6. Baumgart SWL, McLachlan A, Kenny H, et al. Deisolation in the healthcare setting following recent COVID‐19 infection. Viruses 2024; 16: 1131.
- 7. Marinelli T, Ferreira VH, Ierullo M, et al. Prospective clinical, virologic, and immunologic assessment of COVID‐19 in transplant recipients. Transplantation 2021; 105: 2175‐2183.
- 8. Howard‐Jones AR, Arnott A, Draper J, et al. Emergent Omicron BR.2.1 sublineage of SARS‐CoV‐2 in New South Wales, Australia: a subvariant with high fitness but without increased disease severity. Int J Infect Dis 2023; 130: 38‐41.
- 9. Jefferson T, Spencer EA, Conly JM, et al. Viral cultures, cycle threshold values and viral load estimation for assessing SARS‐CoV‐2 infectiousness in haematopoietic stem cell and solid organ transplant patients: a systematic review. J Hosp Infect 2023; 132: 62‐72.
- 10. Alshukairi AN, Tolah AM, Dada A, et al. Test‐based de‐isolation in COVID‐19 immunocompromised patients: cycle threshold value versus SARS‐CoV‐2 viral culture. Int J Infect Dis 2021; 108: 112‐115.
- 11. Razonable RR. Protecting the vulnerable: addressing the COVID‐19 care needs of people with compromised immunity. Front Immunol 2024; 15: 1397040.
- 12. Clinical Excellence Commission. Infection prevention and control manual: Acute Respiratory Infections including COVID‐19 for acute and non‐acute healthcare settings. Version 5.0 (Sept 2024). Sydney: Clinical Excellence Commission, 2024.
No relevant disclosures.